Research
Childpage navigation
Please find below the summaries of our most recent research topics:
Full waveform inversion
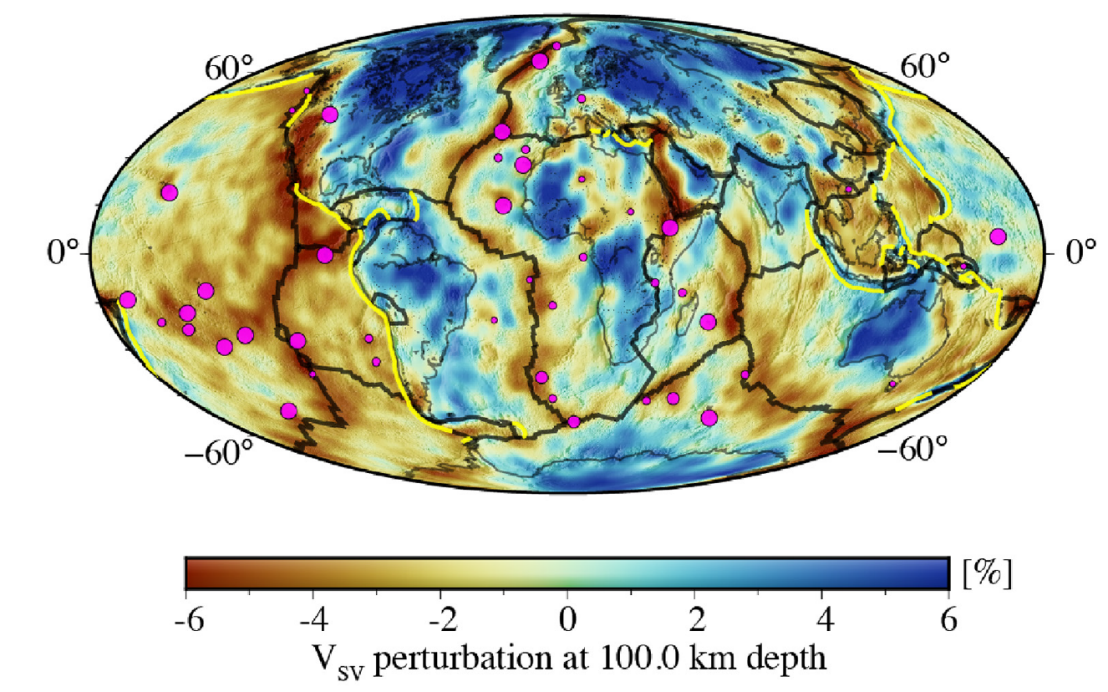
Seismic tomography is among the most powerful tools to explore the 3D internal structure of the Earth. As such, it is key to understand the structure and dynamics of our planet, to constrain earthquake mechanisms, to locate and monitor natural resources, and to model earthquake-induced ground motion.
In our group, we develop new methods for seismic tomography that combine highly-accurate numerical wavefield simulations and adjoint techniques in order to extract as much information as possible from seismic recordings. Furthermore, we apply these methods at a wide range of scales, from medical imaging to whole-Earth tomography.
Most recent publications:
- external page Data-adaptive global full-waveform inversion (external page YouTube video abstract)
- external page Impact of Juan Fernandez ridge subduction inferred from full waveform inversion beneath the South Central Andes
- external page Full waveform inversion beneath the Central Andes: Insight into the dehydration of the Nazca Slab and delamination of the back-arc lithosphere
- external page Evolutionary full-waveform inversion
Fibre-optic seismology
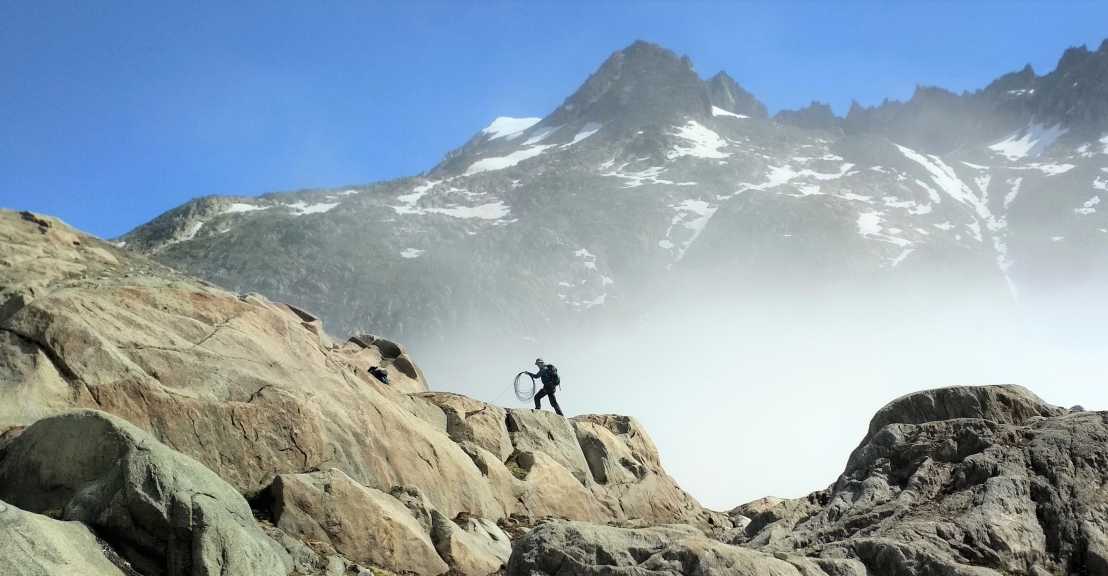
We investigate the use of emerging fibre-optic deformation sensors in seismology. Our specific interest is in applications where dense arrays of conventional seismic sensors are impossible or difficult to deploy. This includes active volcanoes, glaciers, avalanche-prone slopes and dense urban centres. Our research shows that the high spatio-temporal resolution of fibre-optic sensing greatly increases the number of detectable earth- or icequakes and also leads to a significant improvement in location accuracy. In addition to more established Distributed Acoustic Sensing, we study newly developed sensors based on fibre-optic phase transmission.
Most recent publications:
- external page Sensitivity kernels for transmission fibre optics
- external page Theory of phase transmission fibre-optic sensing
- external page Sensing Iceland’s most active volcano with a «buried hair» (external page YouTube field work impressions)
- external page Distributed Acoustic Sensing in volcano-glacial environments – Mount Meager, British Columbia (external page YouTube field work impressions)
- external page Distributed acoustic sensing of microseismic sources and wave propagation in glaciated terrain (external page YouTube field work impressions)
- Download Empirical investigations of the instrument response for Distributed Acoustic Sensing (DAS) across 17 octaves (PDF, 1.8 MB)
The Collaborative Seismic Earth Model
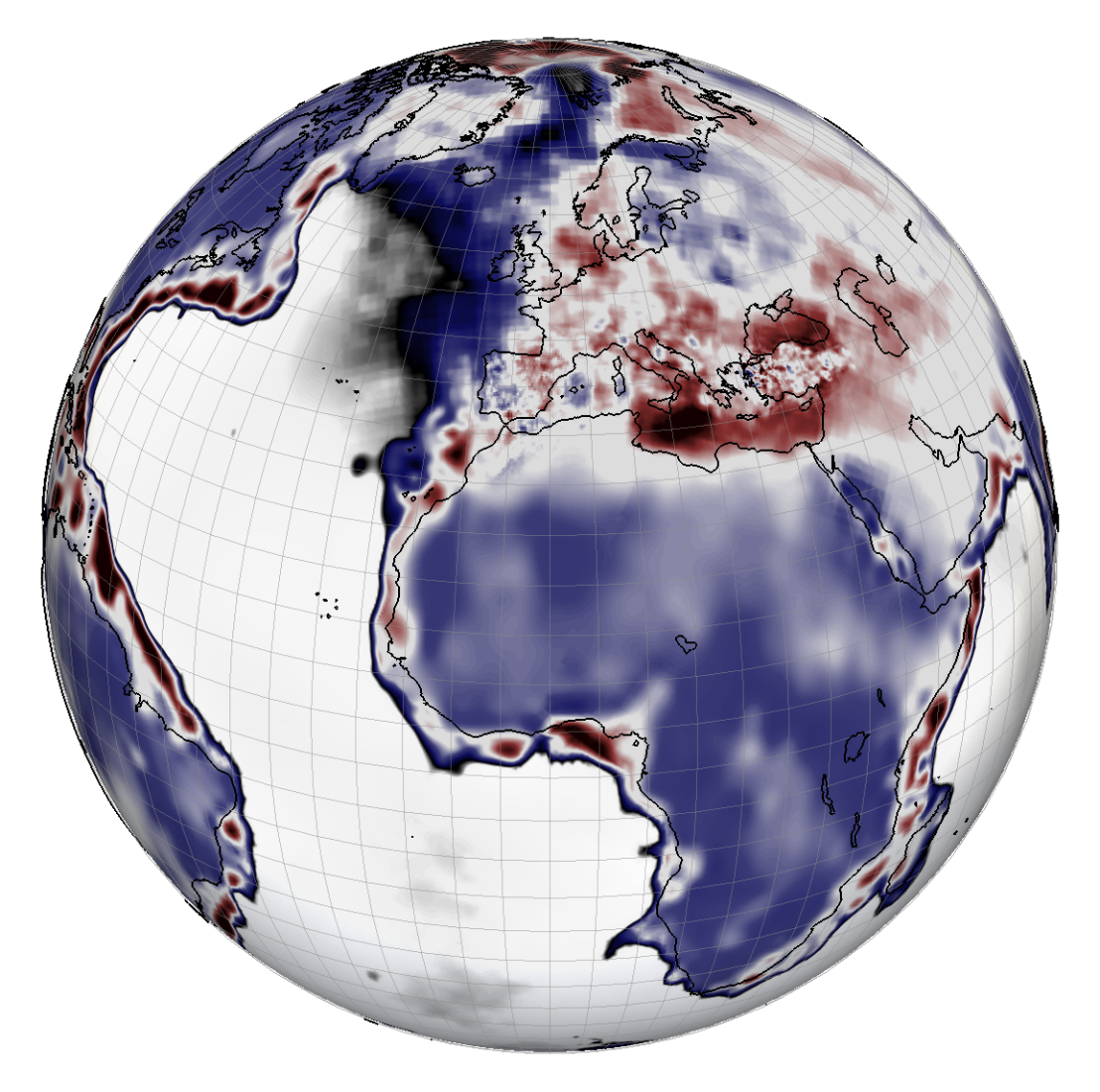
The Collaborative Seismic Earth Model (CSEM) project aims to go beyond the current mode of operation in seismic tomography where individual researchers work independently, thus being limited by their own human and computational resources. Still being in its infancy, the CSEM is a multiscale Earth model that evolves through successive regional refinements, for instance, when new data for a specific region become available. The CSEM framework allows seismic tomographers for the first time to systematically join forces in the discovery of Earth structure and to fully exploit prior knowledge for the benefit of improved resolution. The first-generation CSEM is part of the external page IRIS Earth Model Collaboration. The second generation will become available soon.
Most recent publications:
Numerical wave propagation and HPC
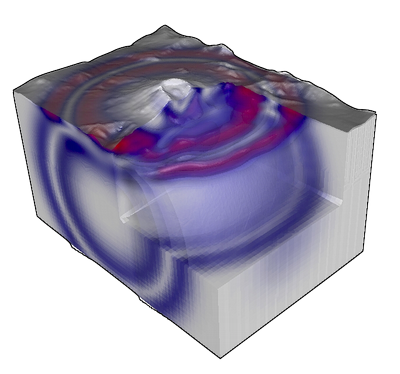
We develop high-performance computing tools for the simulation of seismic wave propagation at scales ranging from few centimetres (e.g., for medical imaging and non-destructive testing) to the whole Earth. For this, we investigate scalable I/O solutions and the use of emerging hardware. A close collaboration exisists with our ETH Spin-Off external page Mondaic that offers user-friendly wavefield modelling and inversion tools for both academia and industry.
Most recent publications:
- external page Accelerating numerical wave propagation by wavefield-adapted meshes, Part II: Full-waveform inversion (external page YouTube video abstract)
- Download Modular and flexible spectral-element waveform modelling in two and three dimensions (PDF, 6.3 MB)
- external page Seamless GPU acceleration for C++ based physics with the Metal Shading Language on Apple's M series unified chips
- Download Fully parallel mesh I/O using PETSC DMPLEX with an application to waveform modelling (PDF, 8 MB)
Ambient noise seismology
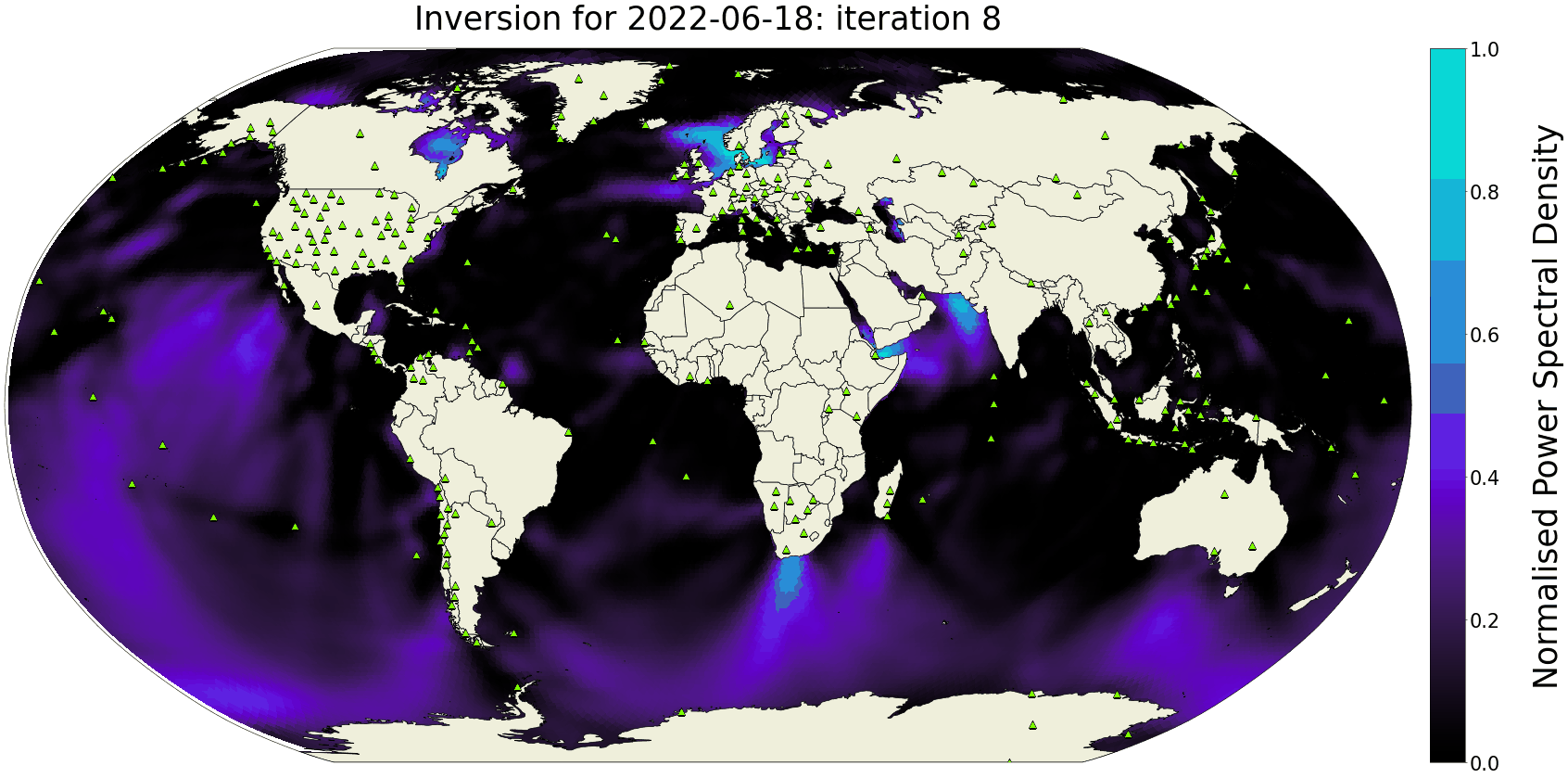
Seismic noise interferometry exploits the ambient seismic field, i.e. the Earth's seismic background radiation, in order to (1) constrain the mechanical interaction of the oceans with the solid Earth, and (2) the 3D structure of the Earth's interior. Traditionally based on the assumption that the sources of the ambient wavefield are nearly homogeneously distributed, we develop new methods for seismic noise interferometry that incorporate more realistic heterogeneous sources that change over time. These methods are intended to joint constrain the sources of the ambient wavefield and Earth structure, thereby leading to improved resolution of both. Applications include the monitoring of subsurface structure, e.g. of reservoirs, even when the ambient field sources changes rapidly over time.
Throughout the past few years, we developed methods and a web application that provides maps of noise source power-spectral density in the oceans on a daily basis: SANS.
Most recent publications:
- external page Seismic Ambient Noise (book)
- Download Rapid finite-frequency microseismic noise source inversion at regional to global scales (PDF, 6.7 MB)
- external page Connecting beamforming and kernel-based noise source inversion (external page YouTube video abstract)
- Download Optimal processing for seismic noise correlations (PDF, 6.9 MB)
- external page Global-scale full-waveform ambient noise inversion
Inverse Theory
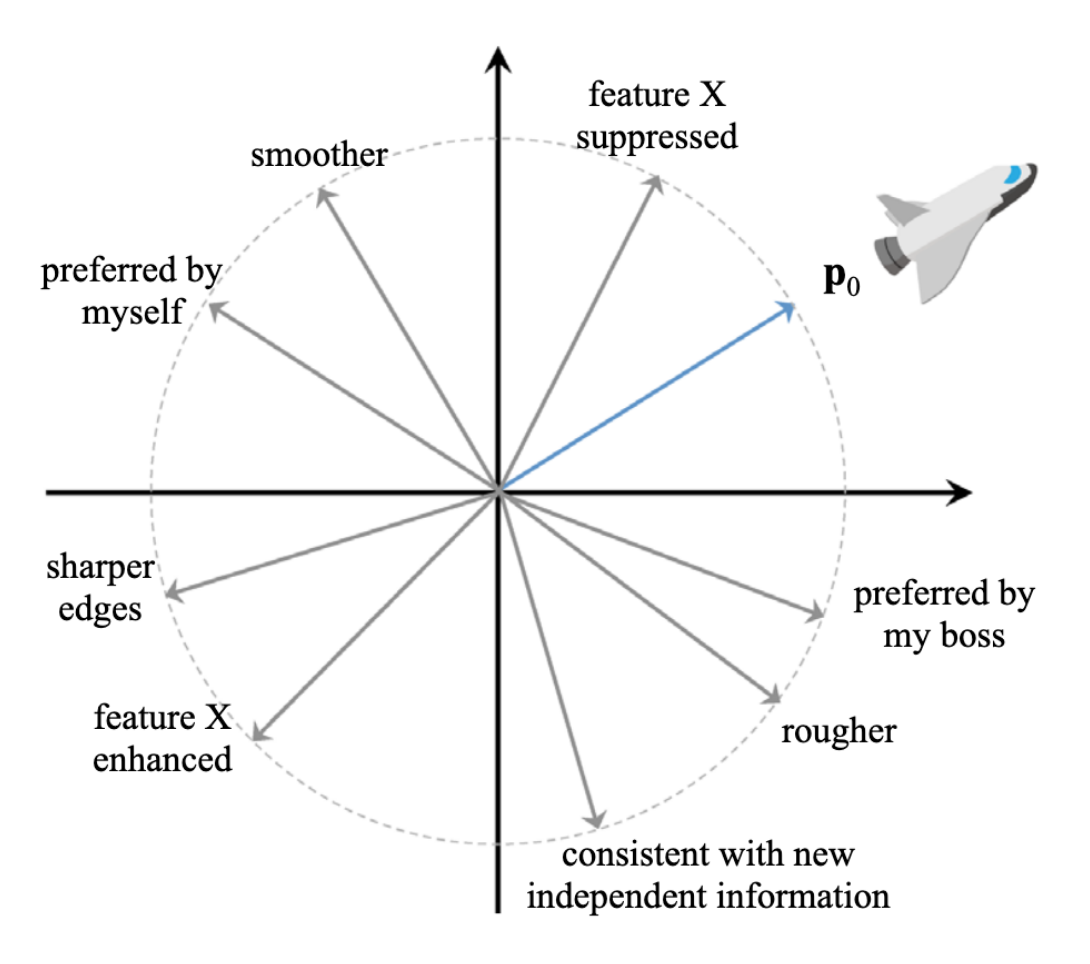
To improve tomographic images and the inferred properties of wavefield sources, we work extensively in the field of inverse theory. Specific topics include Newton-type methods for non-linear optimisation, random probing techniques for uncertainty quantification in full-waveform inversion, Hamiltonian Monte Carlo techniques for the efficient sampling of high-dimensional model spaces, and trade-off analysis in the joint inversion for Earth structure and ambient noise sources.
Most recent publications:
- external page Lecture Notes on Inverse Theory
- external page Autotuning Hamiltonian Monte Carlo for efficient generalised nullspace exploration (external page YouTube video abstract)
- external page Bayesian elastic full-waveform inversion using Hamiltonian Monte Carlo (external page YouTube video abstract)
- Download Hamiltonian Nullspace Shuttles (PDF, 1 MB) (external page YouTube video abstract)
- Download Hamiltonian Monte Carlo Solution of Tomographic Inverse Problems (PDF, 7.9 MB)
Medical ultrasound imaging
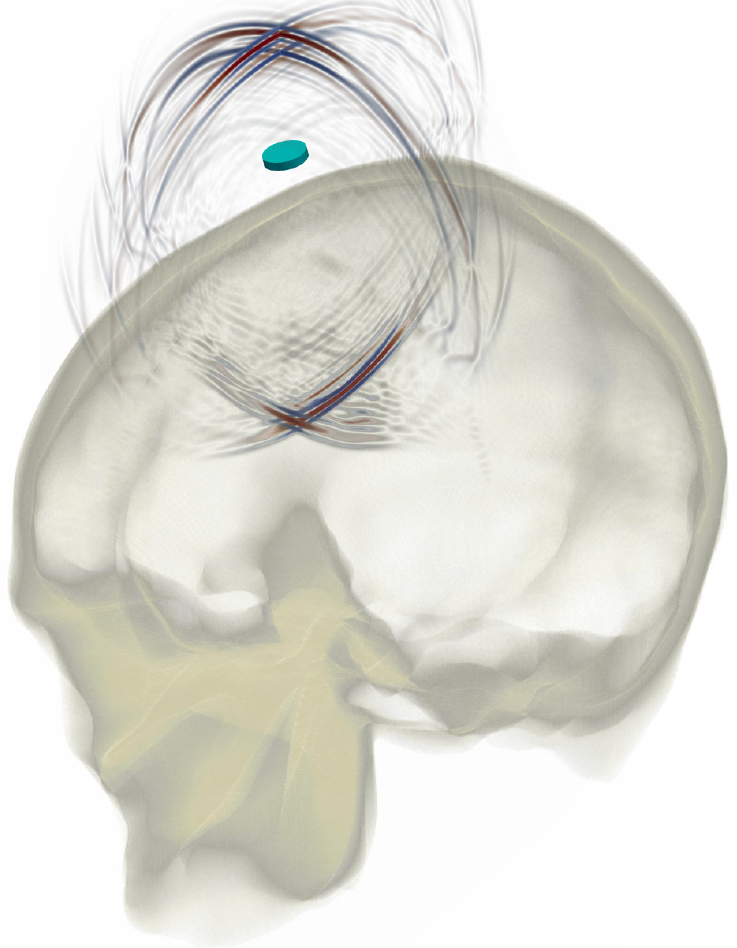
The mathematics and physics of wave propagation are very similar in different application domains, such as seismology, medical imaging or non-destructive testing. This opens opportunities for collaboration and technology transfer.
We actively work on the transfer of seismic imaging and inversion techniques to medical ultrasound. In addition to the adaptation of algorithms used in whole-Earth tomography, this work includes full-waveform inversion for breast cancer detection, the development of active-source random field interferometry for ultrasound, and the modelling and inversion of acousto-elastic wavefields that propagate through coupled fluid-solid media, such as the human head.
Most recent publications:
- Download Diffuse ultrasound computed tomography (PDF, 4.2 MB)
- Download Full-waveform ultrasound modelling of soft tissue-bone interactions using conforming hexahedral meshes (PDF, 4.7 MB)
- Download Analyzing resolution and model uncertainties for ultrasound computed tomography using Hessian information (PDF, 17.5 MB) (external page YouTube video abstract)
- Download Using optimal transport to mitigate cycle-skipping in ultrasound computed tomography (PDF, 4.2 MB)
(Meta)Materials
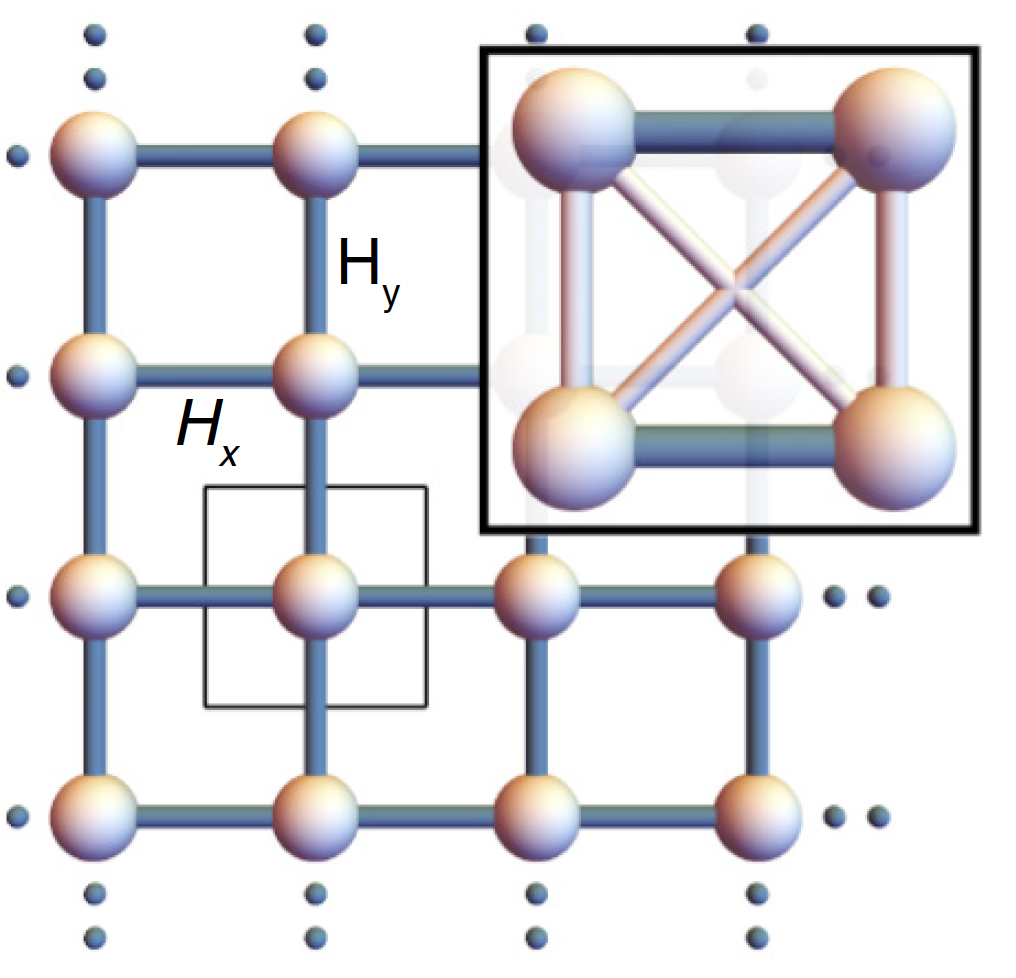
The Earth with all its unresolvable sub-wavelength structure behaves like a large metamaterial. It displays macroscopic properties that differ from the properties of its small-scale constituents, much similar to engineered metamaterials.
Also similar to many engineering materials, the interior of the Earth is not directly accessible. Hence, concepts and practical methods from inverse theory must be employed to learn about the internal structure of such materials without destroying them.
These two aspects establish a close link between seismological research and the material sciences that we aim to exploit in our research. Specifically, we work on the translation of methods and tools from seismology to the metamaterial design and non-destructive testing.
Most recent publications: